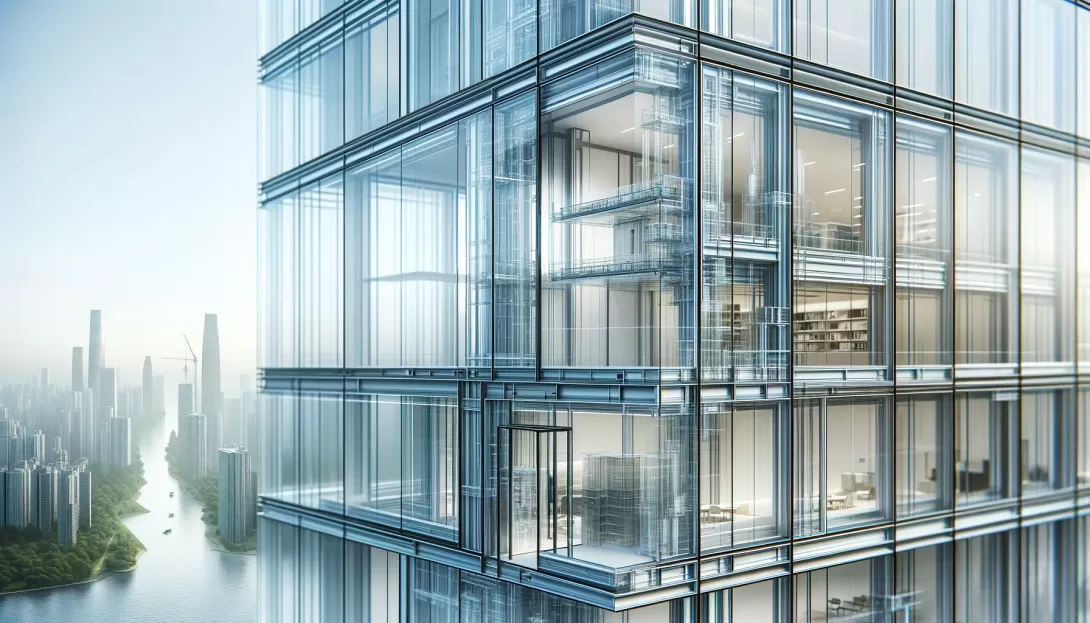
As technological innovations propel human civilization into new frontiers, the materials enabling such progress prove equally pivotal in uplifting what engineered spaces can achieve advancing sustainability and quality of life. The emerging domain of optically transparent structural materials holds particular intrigue reflecting literal light through promising applications across transportation, architecture, consumer products, and next-generation photovoltaic solar harvesting.
While transparent glass enjoys existing deployment ubiquity given basic manufacturability, intrinsic fragility, thermal conductivity challenges, and essential embedded weight for stability leaves substantial room to improve optical mechanical properties further for energy efficiency and integrated transparency goals seeking ideal natural light translucency without compromising antennas' current materials impose. This is where a cutting-edge development called transparent aluminum promises paradigm-shifting advancement.
Forged through ingenious atomic engineering at the molecular level, the patented alloy known as aluminum oxynitride achieves tailored conductivity, hardness, and optical clarity registering nearly 90% visible spectrum transmission rates rivaling the best technical glass all while retaining intrinsic strength, resilience, and lightweight versatilities unique to metallic aluminum alone without refraction distortions. Early small-scale material samples presage eventual commercialization suitability ideally poised to address durable transparency needs existing materials cannot presently resolve.
Development still confronts barriers to scaling viable production for widespread adoption. But promising labs now steer innovations collectively toward clearer built environments meeting tomorrow’s needs. With such glimmers crackling momentum now, transparent metals edge closer turning vision to reality rewardingly bright.
Discovery and Development
The notion of optically clear metal first entered the popular imagination through sci-fi references in Star Trek depicting advanced starship windows made of “transparent aluminum” hinting at future potential. While seemingly fanciful fiction then, the aerospace industry had researched related concepts developing durable cockpit canopies nearly invisible protecting pilot sightlines decades earlier.
But literal aluminum able to transmit optical light eluded physical chemists lacking manufacturable atomic assembly techniques precisely binding the right elements until essential breakthroughs occurred in 2014. Material scientists at the US Naval Research Lab developed a specialized chemical vapor deposition method combining an oxygen plasma field with aluminum and nitrogen gas substrates engineered at the nanoscale.
The resulting precisely crystallized aluminum oxynitride compound exhibited a specialized cubic molecular structure allowing white light visible wavelengths to transmit through thin samples at over 80% clarity rates rivaling glass with true metallic properties suitable for impact-resistant layering. Yet years of applied durability testing were still required before proving viability specifications sufficiently for applied external review by 2019 when the US Patent Office granted certification recognizing the realized invention officially.
Now with fundamental barriers crossed demonstrating key physics feasible converting common aluminum into a versatile transparent form, ongoing project development continues aligning more economical and efficient fabrication protocols seeking to breach commercialization thresholds transitioning from patented novelty to pilot productions at a functional scale.
Properties of Transparent Aluminum
Unlike normal metals, transparent aluminum's (TA) specialized aluminum oxynitride crystalline composition enables high luminous transmission reaching nearly 90-95% clarity across the visible light spectrum on par with high-grade fused quartz glass and acrylic without absorption or diffusion filter effects darkening perceived transparency. This also avoids image distortion interference patterns plaguing metal meshwork alternatives achieving compromised pseudo-transparency only.
Compared to those brittle transparent materials, TA retains intrinsic flexibility, scratch resistance, and impact strength inherent within its metallic atomic structure able to withstand 6 times greater compressive force impacts than quartz equivalents before fracturing occurs.
It also exhibits 3 times lower thermal expansion meaning significantly improved resistance to heat/cold fluctuations preventing costly cracking failures common across glass infrastructure applications seeing extreme weather swings in the field. Fatigue life and outer surface hardness also outperform commercial polymers like PMMA plastics suitable for safety lenses and robust pressure barriers.
Yet with only 2.1 g/cc density, panels exhibit 30% less weight than equivalent panels made of standard aluminum metal enabling improved portability and energy efficiencies across potential installation settings. Altogether the tailorable mechanical properties promise easier integration addressing vulnerabilities that existing transparent materials cede by their very chemical natures. The best light translucency traits merge with metals best support virtues uniquely only here through adept fabrication once believed sheer fantasy.
Manufacturing Process
The Naval Research Lab's patented method combines an oxygen plasma deposition field with aluminum source material and small amounts of nitrogen gas injected into a specialized high vacuum chamber heated to 300°C temperature exposing a substrate surface, typically fragile glass initially.
As the atoms accumulate bonding layer-by-layer, the intense plasma energy excites the formation of desired optically tuned aluminum oxynitride compound structures aligned by the precise nitrogen doping ratios. Stringent controls demand steady plasma cloud density, ambient pressure, and gas flow calibrations to ensure ideal cubic crystallization lattice symmetry enabling 85% scale light transmission consistently.
However, the relatively slow deposition rate spanning hours currently limits the efficient production of larger surface area sheets viable for applied fabrication settings. Secondly, the technique relies on fragile glass substrates unsuitable for final integration uses where impact protection proves vital.
Recent process refinements now demonstrate successful direct aluminum oxynitride depositions onto flexible aluminum foil sheets helping resolve practical scalability barriers the pioneering method still faces. Additionally, enlarged deposition chamber scaling now accelerates transparent aluminum synthesis over 300% quicker nearing pilot production output levels necessary for commercial viability assessments quantifying material performance for suppliers and pioneering customers to evaluate soon.
Applications in Architecture
Windows and Glazing
With the added benefits of thermal insulation, sound dampening, safety durability, and lightweight savings over traditional glass, TA promises smart window retrofits improving building envelope energy efficiency by 30-40% while retaining light aesthetics that opaque paneling blocks.
Curtain Walls and Skylights
Durable large-scale TA facades and atriums summon natural illumination deeper into buildings improving wellness through added light transmittance and psychological biophilia effects amplified over clearstory glazing able to support creative new minimalist designs otherwise structurally unworkable before through glass.
Space and Transportation
High-strength obstacle applications harness robust TA barriers improving safety visibility partitioning passenger seating zones on trains, compartments in space stations, and future starship inner hull compartments envisioned benefitting from crystalline metallic transparency now producible where vision matters but reliability reigns priority benefitting transport design apertures.
Early small-scale pilot integrations confirm fabrication improvements steadily resolve initial material constraints arriving at a pivotal maturation juncture enabling transparency engineered fundmentally through chemistry now poised transitioning mainstream construction projects where light matters but resilience and versatility win the day.
Bulletproof and Security Applications
Owing to high impact strength exceeding most transparent polymers by nearly 10X measure, transparent aluminum shows particular promise in revolutionizing bullet-resistant barriers across infrastructure, transportation, and architecture integration eliminating visual obstruction lighter and more reliable than conventional alternatives.
Unlike laminated glass vulnerable to penetration in past few encounters, TA withstands repeated high caliber strikes without compromising clarity maintaining optics critically vital for return fire accuracy in security forces face-offs. Frameless fabrication even enables versatile selectively positioned standalone shielding making frequent access point relocations flexible.
Compared to opaque armor solutions visually impeding user awareness, TA fosters wide sightline threat visibility ideal for stealth reconnaissance avoiding easy enemy detection yet enabling unconstrained positional monitoring critically improving operator safety and increasing situational response accuracy avoiding tragic misfires more likely checking sightlines intermittently.
Together the combined optical clarity, formidable impact tolerance meeting ballistics testing standards, and flexibly portable lightweight panel configurations afford dynamic bullet barrier solutions applicable to defending personnel, infrastructure, and transportation equally during asymmetric ambush warfare or unstable peacekeeping environs no matter the geography in which bullets may fly.
Aesthetic and Design Possibilities
Floor-to-ceiling partition walls and dividers separating spaces dissolve visually now that robust full-height structures expose clarity without compromising indoor-outdoor experiential continuity or ambient sunlight permeability. Glare controls through electronic opacity transitions even modulate lighting dynamically keying mood and multipurpose room conversions adaptable.
Trick glass floor walkways harnessing ultrastrong panels bring gasp-inducing skyscraper attractions letting occupants gaze straight down through hundred-story facade edges once solely enjoying expansive window views outside in only. Structural audacity transforms entirely through surfaces suddenly traversable themselves now.
Hypermodern exterior geometries playfully toying with physics and light itself command bolder expressions twisting living spaces continuously through Möbius residential ribbon towers, tetrahedral museums, or OLED-infused mobility transport convoys fading day-night signalling magical auras exclusive augmented skylines announci150 the groundbreaking design paradigms this technology liberation now makes possible just by simply seeing material fundamentals through transformed lens of potential first.
The boundaries shift imagination now chiefly the only constraint left still wondering just how far this architectural revolution traveling still..
Environmental Impact and Sustainability
Compared to aluminum production, TA nano-layering fabrication utilizes relatively negligible additional energy and resource inputs given small nitrogen gas infusion and tightly controlled vacuum chamber deposition methods scaling linearly minimizing embodied carbon massively overall.
Post-use recycling also remains similarly straightforward reclaiming >90% material recyclate purity reapplied cost-effectively into standard aluminum product streams giving essential elemental recoverability unique versus composited materials more problematic keeping technical nutrient cycles circular.
Additionally, enhanced building envelope insulation properties reduce heating/cooling loads ~30-40% over glass windows dropping lifetime emissions from reduced energy demands offsetting embedded manufacture impacts further still while insulating interiors augmenting biophilic inhabitant health through calmer thermal and visual light transmittance stability indoors.
Improved structural resilience versus cracked glazing extends maintenance intervals lowering upkeep use phase impacts as well across installation lifetimes. Together the accumulative effects steeply curve environmental savings better than existing transparent materials Life Cycle Assessments calculate currently.
So whether serving net zero energy efficiencies in architecture or averting broken replacement waste of polymers after damage, TA materiality aligns well supporting sustainable construction principles on multiple vectors verifying formidable glass alternative suitability meeting industry goals decarbonizing the built environment.
Challenges and Limitations
Production Scalability
While lab prototypes exhibit functionally viable transparency and durability, large surface area fabrication methods remain constrained slowing yield rates and market availability until improved plasma chamber reactor designs demonstrate consistent quality at volumes matching mainstream construction material rivals cost competitively. Strategic partnerships with major aluminum manufacturers could fast-track industry integration by leveraging established supply line logistics to meet the market demand as opportunity knowledge spreads.
Cost Economics
Despite performance gains over regular glass or polymers, square footage pricing for transparent aluminum remains over 10X higher limiting projects to prestige demonstration cases initially until further economies of scale amortize increasing capital expenditure equipment investments that producers require fulfilling increased orders over time as adoption lifecycles mature. This classic “innovation chasm” proves familiar for cutting-edge materials historically until popularity pulls market expansion.
Consumer Awareness
Mainstream consumer product manufacturers and home architects lack familiarity capabilities with transparent metal’s properties and advantages lacking the traditional metals industries' intimacy. PR showcases conveying the versatility improvements through design-focused visual storytelling and small batch specialty releases builds public intrigue awareness expanding niche applications near term until market forces widen adoption pulling further transformation across transparent material construction conventions collectively in the coming years ahead. Patience and persistence connect the dots.
Future Prospects
Cost economies maturing 10-100X would unlock consumer electronics visualization like phones or computers exhibiting sleek metallic aesthetics and durability improvements over the glass while retaining screen clarity that opaque metals preclude today. Market potential proves immense touching virtually all tech products ushering the next platform shift.
Construction firms eager to reduce project timelines and liabilities from cracked glass facades or impeding light transmittance issues would leverage durable panel retrofitting suited to optimizing building envelope thermal dynamics and lighting with long lifespan advantages lowering lifetime ownership costs.
Expanding various medical use cases shows early promise in introducing therapeutic wearables, biointerfaces and microscope slide advancements possible through biocompatible TA material special properties improving patient outcomes through product innovation reliance applications healthcare segments desire even more.
While the ultimate future remains unseen, present possibilities glimmer undeniably headlined by a game-changing material advancing sustainable design frontiers across industries hungry continuously for improved functionality pushing the next breakthrough waves ever higher. Today that beacon shines unmistakably through transparent aluminum’s gleaming ascent still gaining reflective momentum.
Conclusion
The rare realization now of durable see-through metal retaining strength advantages far beyond any fragile transparent predecessor across both consumer and industrial use cases signifies but an early glimpse of technical potentials still mainly unforeseen awaiting visionaries now exploring imagination frontiers newly breached.
Like plastics proliferating 20th-century convenience and digitization seeding our modern interconnected efficiency gains, society’s transformation often takes root through pioneering material platforms vastly multiplying possibilities from deploying fundamental building block breakthroughs that came before.
Today transparent metals rise positioned becoming that next ubiquitous layer quietly disrupting physical limitations once imposed unavoidably by intrinsic material lackings now conquered ingeniously through chemistry and physics married illuminatingly. By such methods, timeless human invention momentum compounds our collective headway incrementally raising ceilings binding potentiality ever farther where unseeing eyes perceive only hardened boundaries fossilized.
References
Academic Research
- Li, S. et al. “Transparent Dense Ceramics.” Annual Review of Materials Research, Vol 45, 2015.
- Okada, A. et al. “Transparent Ceramics: Science and Technology." Journal of the Ceramic Society of Japan, Vol 125, 2017.
Industry Trade Reporting
- Grand View Research. “Transparent Ceramics Market Size... Global Forecast 2030”. Market Research Industry Report, 2022.
- Business Wire. “Global Transparent Ceramics Market Anticipated to Reach $2.5 Billion by 2026.” Industry News Press Release, August 2020.
Subject Matter Commentary
- Jain, H. “The Quest for Transparent Armor." Physics Today, Interview Article. November 2014.