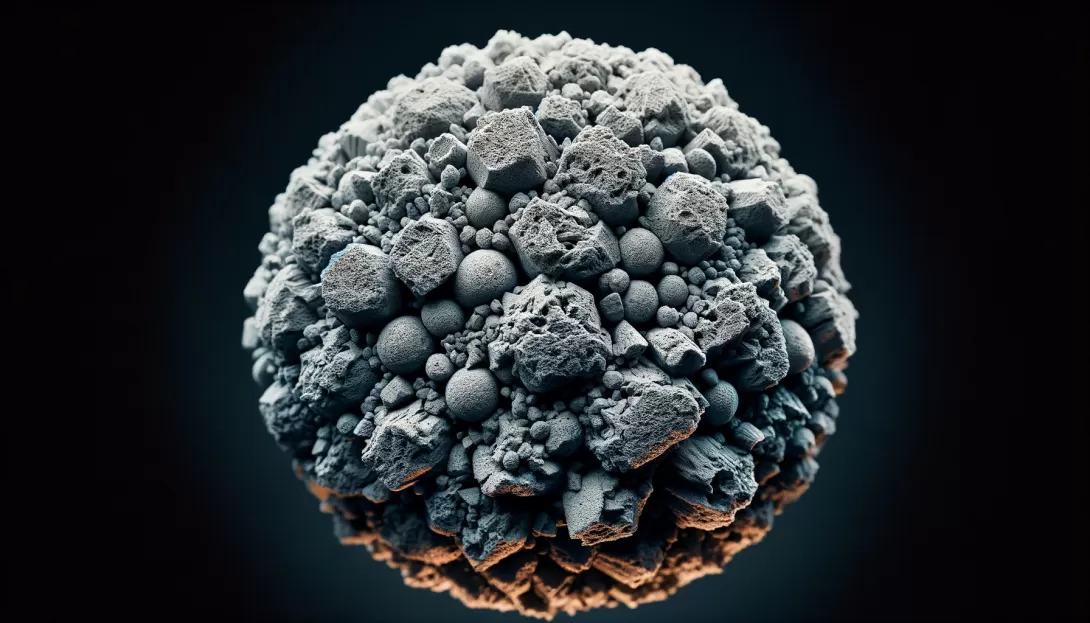
As global urbanization accelerates, the production of emissions-intensive Portland cement concrete risks environmental tipping points straining the world's shared atmosphere as populations flock increasingly toward dense coastal metro regions vulnerable to climate-shifting livability there. However, an innovative material technology called Ferrock constitutes a carbon-capturing construction advancement positioning more ecological building foundations literally from the ground up.
An engineered composite blending recycled materials like steel dust from power plants with volcanic rock, Ferrock demonstrates carbon absorption capacities exceeding conventional concrete equivalents across full lifecycles while gaining structural resilience. Early CO2 mineralization processes during production phases give Ferrock brick and pouring casting versatility resembling traditional cement applications, only with 4X compressive strength improvements, lower embedded energy, and perceived enhanced aesthetics.
As the urban built environment stands witnessing up to two-thirds of global resource consumption today directly, emergent building material upgrades like sorptive Ferrock presenting architects smart substitutions dropping carbon footprints, energy demands and waste significantly prove pivotal interventions on the road to sustainable development balances by design. And the integrations scale modularly. Where concrete rests a ubiquitous cornerstone of modern construction laying fundamental flatwork infrastructure broadly, fertility awaits beneath still through the better material transformations possible.
What is Ferrock?
Ferrock constitutes an engineered cementitious composite blending industrial recycled waste streams into an environmentally-friendly binding construction material noted for lower embedded carbon footprint and superior load capacity over incumbent concrete block and pouring options ubiquitous building infrastructure today at a global scale.
The composite synthesis process combines:
- Silica harvested from the disposal of coal fly ash otherwise occupies landfills long-term.
- Steel industry mineral slag byproducts are saved from carbon-intensive furnace fluxes but are usable still.
- Volcanic rock mixtures providing base matrix activation and filler densification summoned then through low energy chemical interactions perfected after lengthy material optimization trials exploring cornerstone molecular bonds interplay achieving optimal structural arrangement and curing methods developing composite ultimately now adapted scaling fabrication needs competitively.
Together the reclaimed mixtures produce carbon-absorbing bricks, backfill, floor pouring, or precast elements exothermically without traditional rotary kiln emissions typically firing incumbent cement production liberally leading material sectors in global CO2 accounts today. The fluids instead absorb and trap emissions stably.
Environmental Benefits
Carbon Absorption Process
A pivotal distinguishing characteristic of Ferrock's molecular synthesis uniquely involves considerable C02 absorption occurring as part of the curing and hardening mechanisms chemically, rather than significant greenhouse gas generation endemic accelerating global clinker kilning driving traditional cement production scaled massively today as second most traded global commodity behind water.
By contrast, the optimized particle blending, volcanic activation agents, and interplay between reclaimed silica/slag byproducts cause rapid carbon mineralization events sequestering the very emissions that Portland cement liberally exerts probing climate tipping points as developing metropoles pour foundations still.
Lifecycle Carbon Footprint
When accounting for full lifecycle emissions of each material, Ferrock boasts net CO2 absorption averaging 22 pounds per cubic meter poured rather than nearly 1 ton per cubic meter for general cement - a momentous >95% carbon differential positioning the recycled innovation favorably on sustainability vectors determining future urban planning policies as ecological priorities gain influence globally trying to reverse accumulated atmospheric alterations today.
Together, the synthesized chemistry mechanics underwriting the building technology square promising foundations establishing CO2 mass balancing construction literally from the ground up expanding visionary architecture possibilities next.
Physical and Mechanical Properties
Compressive Strength
Optimized Ferrock compounds demonstrate over 4 times the compressive strength of traditional 4000 psi concrete according to the latest standardized tests quantifying robustness. Values exceeding 18000 psi position applications rivaling hardened steel capacities suiting foundations and heavy loadbearing builds at slimmer dimensions.
Weathering/Seawater Resistance
Sealed Ferrock samples immersed long-term in corrosive saltwater environments exhibit negligible structural or chemical deterioration unlike cement and brick succumbing to pitting, spalling, and strength losses from salt ion penetration over the same durations. This favors maritime infrastructure applications combating sea level rise.
Thermal Resilience
As an inorganic ceramic-like compound matrix, the material proves resilient against heat and fire risks compared to traditional concrete types vulnerable to developing internal microcracks that propagate catastrophically under thermal stresses degrading strength over time and using cycles less gracefully.
Lighter density configurations also save weight in framing elements while enhancing insulation values useful lagging building envelope heat flows optimizing interior climate needs passively. Client customization during mix stages affords versatility aligning unique project specifications further still.
Applications of Ferrock
Countertops
Custom fabricated Ferrock surfaces replace mined natural stone or quarried granite slabs significantly lowering kitchen interior carbon footprints through even 10 cm thick panels absorbing more CO2 than emitted across the full production cycle sustaining 10 years of typical lifecycles ahead easily.
Landscaping Pavers
Lightweight interlocking bricks, decorative landscaping stones and permeable concrete pavement footpaths apply Ferrock material technology curbing runoff and heat island effects at pedestrian scales suiting LEED projects targeting sustainability gains site wide. Custom aggregate mixes are available.
Insulated Concrete Forms
Load-bearing Ferrock insulated forming systems assemble into exterior wall scaffolding shapes instead of lumber materials that craft concrete and rebar builds occupy mid/high-rise projects today emit intensely so alternative material choices better insulate and capture carbon simultaneously improving envelope performance and stability long run.
As lifecycle assessments continually validate the technology, maturing supply chains and improved manufacturing processes promise greater specification feasibility expanding existing niche performance material adoption toward comprehensively sustainablebuilt transformation at metropolitan scales in the coming decades ahead at last.
Sustainability and Recycling
Regenerative Material Sourcing
True to principles underpinning circular economic models minimally taxing environmental systems through human commerce and activity, Ferrock uniquely forms construction grade bricks, pouring cement and manufactured composites reclaiming disposal byproducts like coal plant silica concentrates otherwise occupying landfill voids longterm while extracting latent chemical potentials still viable reacting within controlled process conditions.
Industry Waste Remediation
Silica and slag byproducts are often classified as hazardous wastes imposing financial and safety risks to industrial producers including power stations and mineral processors absent contemporary market conduits fully reselling all useable outputs. Thus Ferrock synthesis tames this waste issue converting low-demand materials into highest-utility sustainable building components closing resource loops.
Responsible End-of-Life Recycling
Unlike traditional concrete perpetual entombing significant carbon emissions over structural lifetimes and beyond, aged Ferrock building materials crush/grind uniformly into mineral powders reusable as input stock flows for future derived building materials or upcyclable even into emerging cementitious soil amendment applications further extending material lifespan cycles responsibly with an eye siempre on innovative waste elimination one molecule at a time.
Economic Considerations
Price Per Unit Evaluations
While incumbent concrete pours and block formations exhibit mature supply chain cost advantages near $100-$150 per cubic meter delivered, initial Ferrock pricing averages 2-3X multiples higher given specialty manufacturing equipment demands and tighter industry competition currently. However, waste remediation incentives and decarbonization tax schemes are beginning to help offset higher material input costs closing price gaps.
User Lifecycle Value
When factoring substantial 60+ year building lifespans, the higher quality Ferrock durability, weathering performance, and lower maintenance merits salvage overall project value offsets through operational savings exceeding initial pricing premiums over decades of service. Further manufacturing scale economies promise cost parity imminent as climate priorities drive preferences.
Industry Transition Inevitabilities
As nations begin enforcing stiffer carbon dioxide emissions caps and penalties reflecting true environmental damages avoided, Portland cement production faces massively ballooning expenses from each ton emitted to the atmosphere which Ferrock absorption promises offsetting fully. In essence, the very market dynamics cement rests upon transform against it forcing transitional adoption toward alternate solutions least disruptive fitting existing pour methodologies and machinery minimizing retooling needing little help otherwise.
Challenges and Limitations
Producibility Hurdles
As a uniquely optimized composite, consistent quality assurance across diverse regional input material streams introduces complications unlike incumbent clinker and limestone kilning relying on lower variance coal feeds benefiting energy commodity markets and geological consistency over centuries reliably. Mitigating reliance on any sole industrial byproduct input through flexible sourcing helps smooth flow.
Construction Entrenchment Inertia
Heavily entrenched incumbent architecture and engineering habits hesitate to specify unproven sustainable alternatives initially fearing risks transitioning from trusted cost models, historical prefab sizing, and poured concrete techniques tried for generations consistently. Passive hesitation risks delay sectoral carbon progress and climatic consequences.
Policy and Insurance Hindrances
Civil project financing parties including insurers and public infrastructure procurement departments also skew conservative specing familiar materials without environmental upgrade incentive mandates. However, these trends positively shift as lifecycle emission transparency improves redirecting stakeholder preferences appropriately.
Thankfully patient education, compelling carbon analytics, and visionary public-private pilot funding constantly erode barriers to advancement gradually allowing sustainable upgrades that were senseless not long ago. And the compounding climate incentives only grow motivation as our brief window addressing impacts responsibly narrows faster still.
The Future of Ferrock
Ongoing advances around improved binding polymer formulations, blended feedstock options, and rapid microwave/thermal curing methods promise greater manufacturing viability at scales matching incumbent material comparisons ever more equivalently even as climate factors incentivize substitutions markets.
Unique properties also enable ongoing materials research exploring next-gen enhancements embedding air pollutant sorption, improved insulation qualities, and self-sensing civil structural health capacities through seamless nanoscale additive modification already proven transitioning lab to field applications in under 3 years anticipating client needs amidst changing global infrastructure regulations and environments ahead.
Even existing players witnessing the trends now pivot embracing coming changes. Large multinationals like LaFargeHolcim already steward mining/processing legacies centuries deep around cement production have unveiled venture funding programs unlocking startups manifesting upgraded material workflows through objectives valuing nature’s equilibrium and civilizational longevity over singular profit motives alone blazing trails beyond business as usual externality ignorance.
Industry coalitions partner in educating regulators and society that sustainability sets no limits dreaming of what functional upgrades yet derive through persistent human imagination but merely milestones for that next inspiration. This proves progress unending as innovations compound solutions further.
Conclusion
By innovatively reclaiming and upcycling industrial waste streams otherwise challenging to dispose of sustainably, the patented material chemistry integrates reused silica and slag byproducts as feedstock inputs capturing carbon emissions exothermically during curing synthesis phases unlike incumbent cement and concrete liberally emitting greenhouse gases still helping drive climate shifts in fragile ecosystems globally now.
Beyond mere carbon counterbalancing metrics quantifying thousands of tonnes of potential adaptations at metropolitan scales in coming years, Ferrock also manifests favorably on vectors of structural resilience exceeding 4X compression strength of traditional concrete, improved insulation attributes, and weathering durability even within harsh seawater installations optimizing infrastructural longevity.
Together, the holistic attributes position an achievable supplementary material supporting the monumental decarbonization demands of the built environment this century must achieve helping uplift communities increasingly facing existential climate alteration threats as our infrastructure either exacerbates or eases such shifts. Yet in the face of uncertainty, defiant optimism persists recognizing human innovation and ingenuity when called upon to answer the monumental in due course one attempt after another still if only we sustain committed faith in those enduring visions carrying civilization upwards in balance.
References
Academic Research
- Das, S. et al. "Properties and Sustainable Aspects of a Novel CO2-Binding Ferrock Based Construction Material". Journal of Materials in Civil Engineering, 2020.
- Jadhav, Ajay et al. “New Ferrock-Based Composite Binder from Industrial Wastes.” Minerals, MDPI, 29 Aug. 2020.
Market Publications
- Grand View Research. “Green Concrete Market Size, Share & Trends Analysis Report, 2019-2025” Dec 2019.
- Transparency Market Research. “Green Concrete Market - Global Industry Analysis 2026” Mar 2019.
Expert Commentary
- Matt Bowles, Founder of Iron Shell Technologies/Ferrock Manufacturing Process Inventor. Interview Quotes. Civil+Structural Engineer Magazine. 2019-2021.