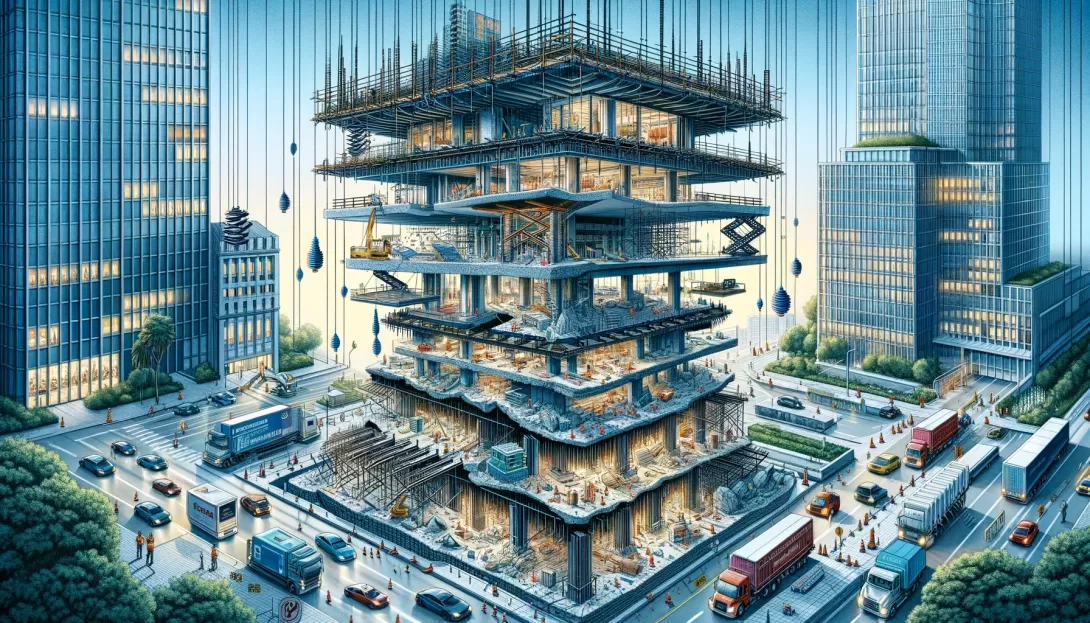
Seismic retrofitting refers to the structural modification of existing buildings to improve their earthquake resilience and safety. It involves assessing vulnerabilities in a building's design and then adding structural bracing, reinforcements, isolators, or dampers to strengthen resistance to seismic activity.
Undertaking seismic upgrades proves critical for properties located in earthquake-prone regions globally, from Pacific Rim nations like Japan and New Zealand to coastal California in the United States to Mediterranean countries like Greece and Italy. Earthquakes frequently cause billions in damage to buildings never designed according to modern seismic codes, resulting in collapse risks threatening inhabitants.
Common seismic deficiencies include inadequate lateral load resistance, weak foundations, irregular building geometries, poorly braced connections and structural framing, and heavy overhanging stories. Targeted retrofits address site-specific vulnerabilities proactively before the next major quake, thus boosting community resilience and reaping substantial life safety and economic rewards over time.
Understanding Seismic Activity
Grasping why and how earthquakes damage buildings informs effective seismic mitigation approaches. Major geologic processes and events highlight retrofitting’s vital role in resilience:
Seismic Waves
The ground shaking from seismic waves places intense lateral and vertical loads on structures as fault ruptures rapidly release pent-up tectonic energy. Shear waves prove especially destructive for buildings.
Building Movement
A rigid building foundation on turbulent subsoil leads to differential building distortions from the waves, stressing vulnerable structural connections not designed to flex without fracturing. Walls separate from floors leading to partial collapse.
Past Earthquakes
Event analysis validates retrofit value. For example, modern updates prevented catastrophic pipeline fires during Japan’s 2011 quake. New Zealand’s stringent retrofit policy has continually strengthened its resilience since 2011.
Takeaways include bracing buildings for applied lateral loads via shear panels or frames while adding damping mechanisms, as well as improving continuity between floor and wall components enabling unified deformation without outright failure. These together satisfy the overarching seismic objective: structurally dance in harmony with the shaking earth!
Principles of Earthquake-Proof Engineering
Several fundamental concepts guide engineering buildings to withstand intense ground shaking and associated stresses:
Ductility - Structural connections and materials that can plastically deform under cyclic loads allow controlled energy dissipation without immediate failure, enhancing survivability.
Lateral Stiffness - Braced frames, shear walls, and core structural elements resist racking from applied horizontal inertia loads preventing collapse while allowing some elastic sway.
Isolation - Base isolators including dampening bearings, pads, or pendulums functionally decouple buildings from severe ground distortions for reduced accelerations throughout structures.
International model building codes have continually raised minimum standards for inelastic deformation and serviceability following intense seismic activity based on simulation research. They set requirements on load path continuity, drift limits, ductile detailing, irregularity restrictions, and seismic force minimums calculated from site intensity and building occupancy. Adherence to sound codes enhances resilience.
Techniques in Seismic Retrofitting
Numerous retrofitting approaches are tailored to the deficiencies, construction methods, materials, and use of existing properties:
- Base Isolation Retrofits: Concrete or rubber damping pads inserted between foundations and buildings filter ground vibrations. Braced sliders also attenuate motions.
- Supplemental Damping Devices: Added friction, viscoelastic, or metallic-based dampers absorb seismic energy imposed on structural elements yet remain unfazed themselves if properly anchored.
- Shotcrete Reinforcement: Steel or carbon mesh jacketing beneath sprayed concrete overlays strengthens columns and structural walls through composite confinement effects.
- Masonry Wall Bracing: Surface-bonded composite fabrics paired with metal anchors or concrete shear elements brace brittle brick walls prone to out-of-plane failures, maintaining integrity.
- Wood Structure Tying: Using metal anchor ties and straps, continuous load paths improve weak connection points between wooden elements vulnerable to splitting and debris impalement.
When tailored to building types using optimal mechanisms and thorough inspection, installations significantly boost site resilience. Ongoing maintenance of upgrades remains necessary.
Assessment and Planning for Seismic Retrofitting
Vulnerability Assessment
Trained structural engineers audit localized hazards, structural typologies, ages, conditions, and occupancy uses onsite to gauge relative vulnerabilities against intense projected shaking and collapse/damage risks. Historical data informs predictive models.
Cost-Benefit Analysis
Projected seismic intensity models and assessed vulnerabilities reveal maximum probable building repairs and failure consequences. Compared to planned retrofit expenditures, ideal upgrade scopes and cost efficiencies emerge. It guides resource allocation.
Scope Prioritization
Multi-building portfolios stage phased upgrades first target the most vulnerable structures, mission-critical operations, and dense occupancies, as well as low-cost, high-impact interventions like securing building appendages. This logical sequencing stretches capital most effectively.
Policy Incentives
Governments often provide financial incentives like tax rebates to facility owners to undertake voluntary retrofit initiatives aligned with public risk reduction goals. This accelerates upgrades. Mandating upgrades upon sale also helps over time.
Planned, informed, and incentivized seismic hardening programs best support communities structurally defend against geological hazards, saving lives and stability.
Materials and Innovations in Seismic Retrofitting
Shape Memory Alloys
Metals like nickel-titanium dissipate seismic energies across lots of deformation cycles. They also self-center structures from lateral drifts. Minimal bulk makes them adaptable to structural reinforcement.
Tuned Mass Dampers
Custom-weighted moving inertial bodies mounted within buildings counteract resonant vibrations. Spring-loaded sliding plates now automate and optimize damping through real-time motion tuning.
Carbon Fiber Composites
High-strength, moderate-stiffness carbon fabrics bond onto many structural substrates. This overlay arrests cracks and adds shear resistance with little incremental mass. UV-hardened resins suit field installations.
Real-Time Stability Monitoring
Dense networked lidar, camera, and MEMS sensor arrays combined with structural analysis software track building movements and flag excessive drifts or accelerations triggering automated hazard responses like fire suppression or elevator recall.
By blending material science innovations with digital stability enhancements, next-generation retrofits promise the precision, adaptively responsive seismic protections scaling across urban infrastructure. Resilience gains happen proactively.
Benefits of Seismic Retrofitting
Beyond preserving real estate assets and critical building functions, strategic seismic hardening delivers far-reaching rewards that affirm retrofitting as a wise public good investment:
Life Safety Assurances
Upgraded structures better shield vulnerable populations from avoidable injury and loss of life throughout communities. This benefit remains incalculable.
Avoided Repair Costs
Each dollar invested in retrofits conservatively reduces quake repair expenditures by $4. Insurance premiums also gradually decrease for proactive owners as actuaries factor lowered risks from proven countermeasures.
Historic Preservation
Securing heritage masonry buildings with sensitive structural reinforcements maintains cultural continuity through emblem landmarks downtown. Unique architecture persists in welcoming tourists.
Community Resilience
With adequate buildings remaining operational after events to host recovery crews, shelter displaced people, and restart business activities essential for coping and rebounding, whole towns lift faster collectively back to normal.
Rewarding far beyond the properties themselves, seismic retrofits thus epitomize socially responsible preparedness for interconnected communities facing shared geologic futures.
Challenges and Considerations
Project Expenditures
Full building retrofit costs can approach $100 per square foot, challenging budgets. Owners require creative assistance like engineering analysis subsidies, tax breaks on upgrades, and resilience bonds supporting sequential multi-year community upgrades.
Preservation Hurdles
Modifying heritage buildings risks architectural damage or alterations diminishing historical visual integrity. Careful structural overlay solutions, like discrete wall plating or isolated foundation buffers uphold aesthetics amid upgrades. Policy intricately balances factors.
Compliance Ambiguity
Local seismic codes often grandfather existing buildings without requiring legally mandated upgrades as land usage and occupancy continue unchanged over time regardless of shifting seismic data. Trigger events for mandatory action based on cost-effectiveness merit debate.
While seismic preparedness proves beneficial, tailored regulatory measures and funding mechanisms are key to equitable access alongside flexibility upholding public safety without undermining community culture or economic stability emerge through inclusive disaster resilience planning.
Case Studies
Categoric evidence proves careful seismic retrofitting reliably saves structures across risk zones, especially when approached holistically:
Mexico City Hospital
Severely damaged in the 1985 quake, a staple 1940s tower was further reinforced with a cradling exoskeleton dissipating seismic energy through replaceable fuses, retested in 2017's deep epicentral tremors with only minor nonstructural damage. Holistic resilience surpassed life safety to fully operationality.
New Zealand Parliament
After enduring 0.7g accelerations in 2011 plus 10,000+ aftershocks unscathed thanks to base isolators installed in 2005, further hardening now protects the entire Wellington harbor’s cultural icons like national museums. Proactive policy spares irreplaceable heritage.
Los Angeles High-Rises
Following 1994's Northridge earthquake unveiling key welded beam defects, LA’s city hall among other buildings had critical connections retrofitted with malleable joints meeting upgraded codes preventing fractures seen in towers built even decades later during distant quakes. Regulation catalyzes stock resilience.
When seismic risk realities align stakeholders and sufficient budgets around resilience goals for important community spaces, successive generations enjoy cohesive cultures laced with continuity.
The Future of Seismic Retrofitting
With densifying cities facing growing seismic hazard exposure, research and development accelerating future-forward techniques promise more preemptive, integrated safeguards:
Widespread Sensor Infrastructure
Pervasive structural monitoring networks track accumulating stresses citywide, flagging unseen vulnerabilities while also tracing building motions during actual tremors in consideration for damage-responsive emergency systems.
AI-Assisted Retrofit Design
High-throughput simulations assessing millions of possible reinforcement schemes for maximized post-quake reparability and operational continuity better inform selective upgrade investments using cloud computing.
Standardized Modular Retrofits
Factory-built bolt-on isolation and damping "retrofit kits" tailored to common building types simplify custom field installations. Economies of scale drive down adaptation costs as takeup accelerates.
Ultimately seismic science progresses toward not only life-critical stability but operational resilience - enabling buildings and infrastructure to suffer only minor performance losses when the earth roils. Blending sensing, data analytics and manufacturing advancements accelerate stronger yet affordable seismic countermeasures.
Conclusion
In conclusion, seismic retrofitting crucially bolsters community resilience and public safety as the world’s earthquake hazards remain unavoidable.
Carefully assessing vulnerabilities and then tailoring structural reinforcements and control systems to shore up weaknesses has spared innumerable lives historically while enabling fuller, faster recoveries societally. Prepared structures also better preserve economic continuity and cultural heritage through environmental turbulence.
Yet colossal untapped opportunity remains regionally to implement technical best practices at scale guided by policies that incentivize seismic responsibility. Increased insurance incentives, streamlined engineering subsidies, and Codes modernizing around outcome-based benchmarks over outdated prescriptions will further progress.
Ultimately, championing resilience is an intergenerational undertaking requiring sustained, widespread commitment financially and culturally. As threats loom, modern societies dismissing seismic risks lose more than brick and mortar long-term; instability cracks continuity. Thus grounding communities requires retrofitting foundations - physically and metaphorically. The task beckons tireless vision and vigilance.
With ongoing diligence and care for entire communities’ safety, seismic retrofitting promises to enable societies to persevere through the Earth’s awesome seismic power.